Venus Tectonics Look Like Pack Ice
By Paul Byrne
Earth’s nearby neighbor seemed inactive, but new maps and models have exposed its complex volcanic and crustal deformation surface features.
July 12, 2021
The Long View Astronomy Astrogeology
Editors’ note: Paul Byrne wrote the January-February 2021 cover feature on Venus, and this research updates what he reported in that article.
For many years following the end in 1994 of NASA’s Magellan radar-mapping mission to Venus, most planetary scientists regarded the Solar System’s second planet as a relatively inactive world, its geological quiescence perhaps punctuated by occasional, catastrophic outpourings of lava. Some circumstantial evidence pointed to ongoing volcanic activity, but the surface revealed by the Magellan showed no trace of the global mosaic of tectonic plates that characterizes Earth. Much of the planet appeared covered by basaltic lavas that erupted within the last several hundred million years, in stark contrast to the dynamic surface of Earth.
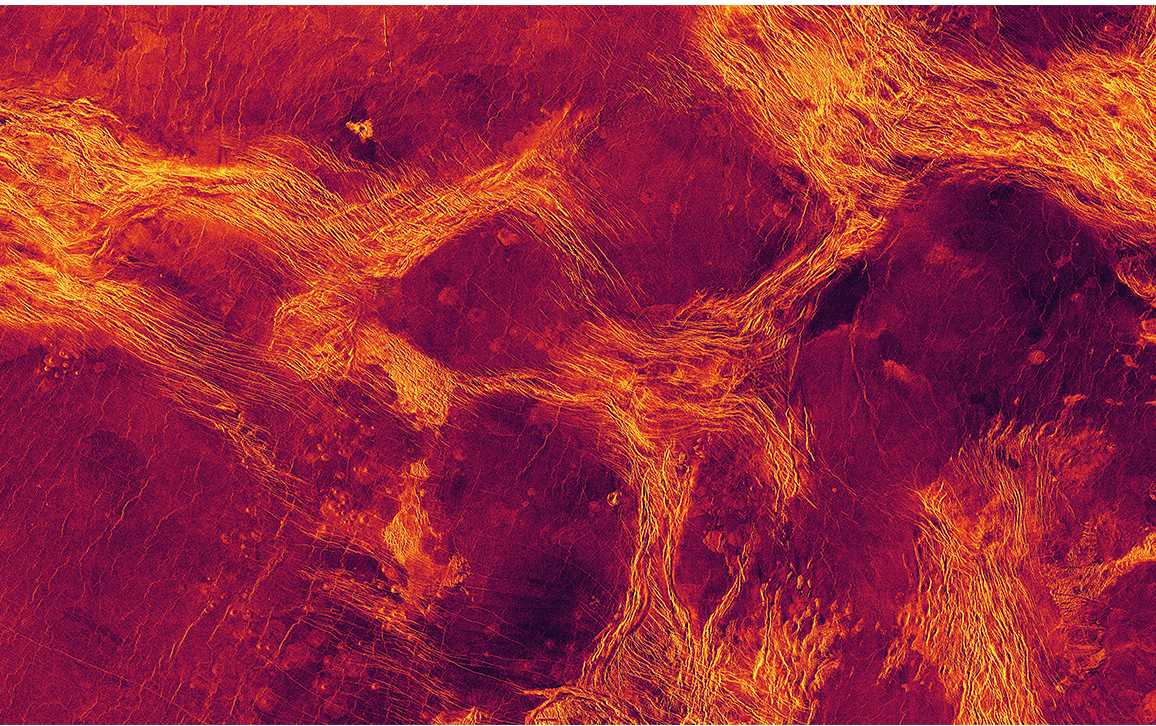
Paul K. Byrne/NASA/JPL-Caltech.
But that view started to change in recent years, in part because of additional evidence of recent volcanism from the Venus Express spacecraft of the European Space Agency (ESA) in the 2010s, and in part because of continued work to map, model, and understand the complex volcanic and tectonic features across the Venusian surface. One hypothesis that had yet to be fully investigated was whether the forces from mantle convection could cause deformation at the surface—in a manner somewhat similar to what drives Earth’s plate tectonics engine. But to what extent, and where, that process operated on Venus, assuming it operated at all, remained wholly unclear.
My colleagues and I tested this hypothesis by analyzing Magellan radar image data, searching for and mapping areas of substantial tectonic deformation. Our goal was to look for evidence, preserved in the morphology and distribution of tectonic structures, of surface deformation that could be attributed to motion within the planet’s interior. As a reality check, we also investigated whether mantle motion would be able to deform the surface in the first place by running models of mantle convection based on gravity field data returned by Magellan.
What we found was surprising. Sure, scientists had known for decades that Venus is heavily tectonically deformed, with some of that deformation concentrated into narrow bands of tectonic structures. But we recognized, for the first time, that in many places these bands intersect in such a way as to delineate low-lying areas that themselves show very little tectonism. And whereas earlier studies had found localized examples where Venus’ crust had shifted side to side, we were able to show that this style of deformation was remarkably common. In short, we’d pieced together individual lines of evidence to build a picture of the crust having been fragmented into individual blocks that have rotated, moved, and jostled together akin to how pack ice moves atop a frozen lake.
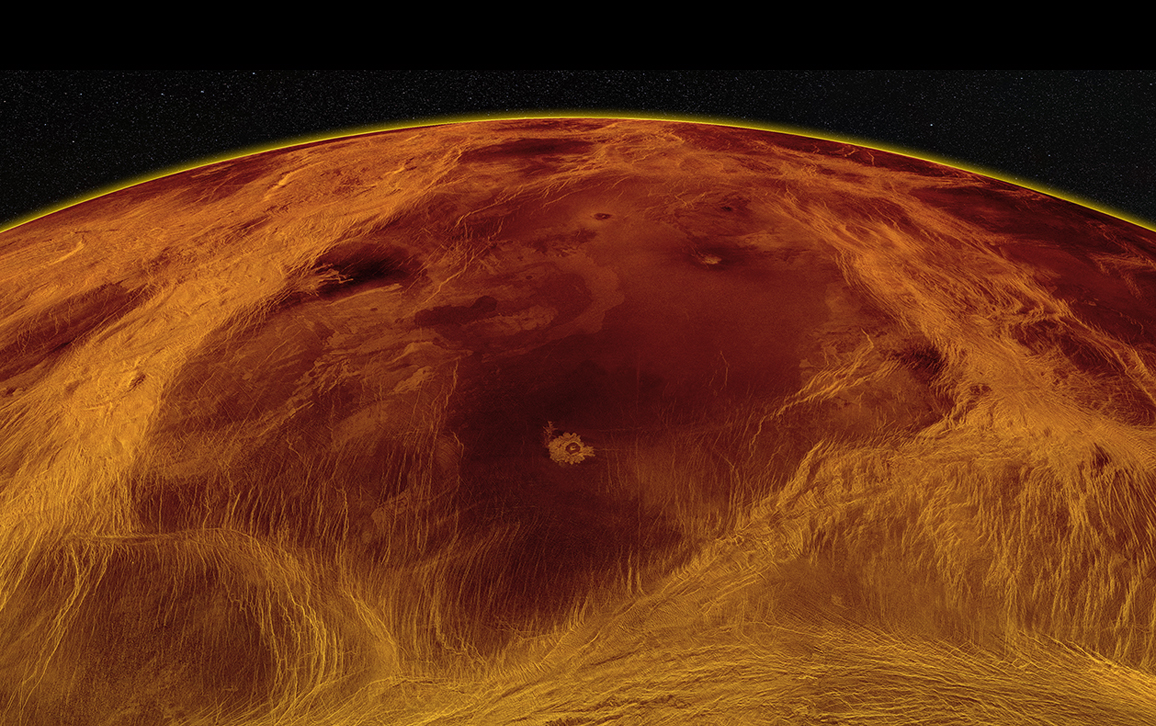
Paul K. Byrne/NASA/JPL-Caltech.
Such jostling of crustal blocks isn’t seen on the surfaces of Mercury, the Moon, or Mars, but it is similar to how blocks of continental crust move on Earth. A global survey of Venus showed these blocks to be relatively modest in size (the largest is the size of Alaska, the smallest only a couple of hundred kilometers across), but widespread. They are distributed across much of the planet’s lowlands, which occupy about 70 percent of the surface. And intriguingly, some of these block-bounding tectonic structures cut through geologically young lavas, suggesting that at least some of this jostling of the crust has taken place relatively recently. (Establishing how recently these events happened on Venus is challenging. But even if the activity took place tens of millions of years ago, it seems unlikely that the geological engine of Venus—a planet almost as big as Earth—would have shut down in just the most recent one percent of its history.)
Our mantle-convection models filled in an additional, critical piece of this puzzle: For the best estimates we have of the composition of Venus’s crust, the strength of the lower part of that crust is weaker than the forces we calculate for mantle motion at every location where we see the block pattern. These results thus give us a mechanism for how the individual blocks form: The slow but powerful roiling of the mantle, heated from below and forced to convect like water in a boiling pot, applies sufficient stress to the overlying crust to fragment it and shift around the resulting “pack ice” pieces.
Taken together, our findings support the hypothesis that Venus’s interior has deformed the surface in the recent past, and probably continues to do so, in a way that’s not all that different from the way that tectonic plates move horizontally on Earth. There are key differences: Although Earth’s oceanic plates are probably similar in composition to the crustal blocks on Venus, Earth’s plates are cooler and more rigid, which allows them to subduct into the deep interior in a process that helps regulate the temperature of our planet. On Venus, the crustal blocks jostle but they are too weak to subduct, and so remain trapped at the surface. And, despite similarities in how they bump and shimmy, the blocks on Venus are compositionally very different from the continental crust on Earth.
Our findings add to the growing evidence for Venus being geologically alive, and perhaps not all that dissimilar to Earth, even if at face value these planets seem worlds apart. To test our hypothesis of a fragmented and mobile crust on Venus, we will need radar image and topographic data at higher resolutions than afforded by Magellan. Fortunately, NASA and ESA recently announced that together they will send three spacecraft to Venus by the end of this decade, which will provide a vastly improved picture of the planet’s complicated tectonics. In doing so, we will come to better understand not only our enigmatic planetary neighbor, but what kinds of outcome we might expect for large rocky planets generally. As we discover ever more such worlds in orbit around other stars, we will need to learn whether the plate tectonics, oceans, and long-term habitability of Earth is the rule—or the exception.
American Scientist Comments and Discussion
To discuss our articles or comment on them, please share them and tag American Scientist on social media platforms. Here are links to our profiles on Twitter, Facebook, and LinkedIn.
If we re-share your post, we will moderate comments/discussion following our comments policy.