Going Rogue with Optical Waves in the Laboratory
By Gregory J. Gbur
New optical research suggests that rogue waves, which can be higher than a 10-story building in the ocean, can form more easily than once thought.
October 21, 2015
Macroscope Physics
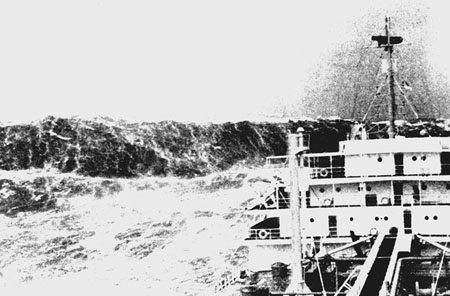
Image from Wikipedia
One moment in the deep blue wave,
The next upon the topmast spray,
The winds weave riband as they rave,
Long lines of foam that glide away.
Then sweeping o'er the Xebec's side,
A monster wave pours crashing in,
No bark could brave the sea's blue tide,
Spares not the crew the rolling din.
—From The Xebec, William Ellery Channing (1843)
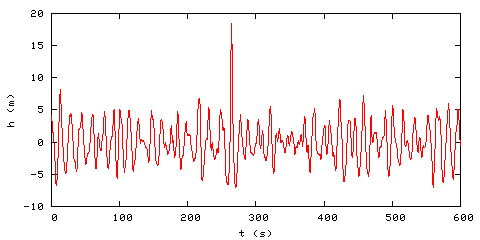
Image from Wikipedia
For generations, sailors have told stories of gigantic, ship-breaking waves appearing seemingly out of nowhere, even in calm seas. Such waves, now known as rogue waves, did not come to the attention of science until New Year’s Day 1995, when a massive 60-foot wave slammed into the Draupner natural gas platform off of Norway’s coast. The height was recorded by laser sensors and confirmed by damage to the platform, and since its observation researchershave discovered that such monsters are distressingly common. One satellite survey in 2004 saw 10 giant waves over 81 feet high during a three-week period.
Until these discoveries, such rogues were thought to be so incredibly rare as to never be encountered. Now they are recognized as a genuine threat to ocean-going vessels, and perhaps one of the leading causes of ships being lost to Davy Jones’s Locker. But what causes them? There are a number of factors that are thought to possibly contribute to their formation, but it is unclear how important each of these factors is. Unfortunately, studying such waves in their natural environment is simply not possible, due to their relative rarity and unpredictable appearance.
This has presented a unique opportunity for optics researchers, who for nearly a decade have been investigating the formation of rogue waves in the laboratory using light. This August, a research collaboration including scientists from France, Ireland, and Finland reported on the creation of an “optical sea” exhibiting rogue waves, using a simple optical system.
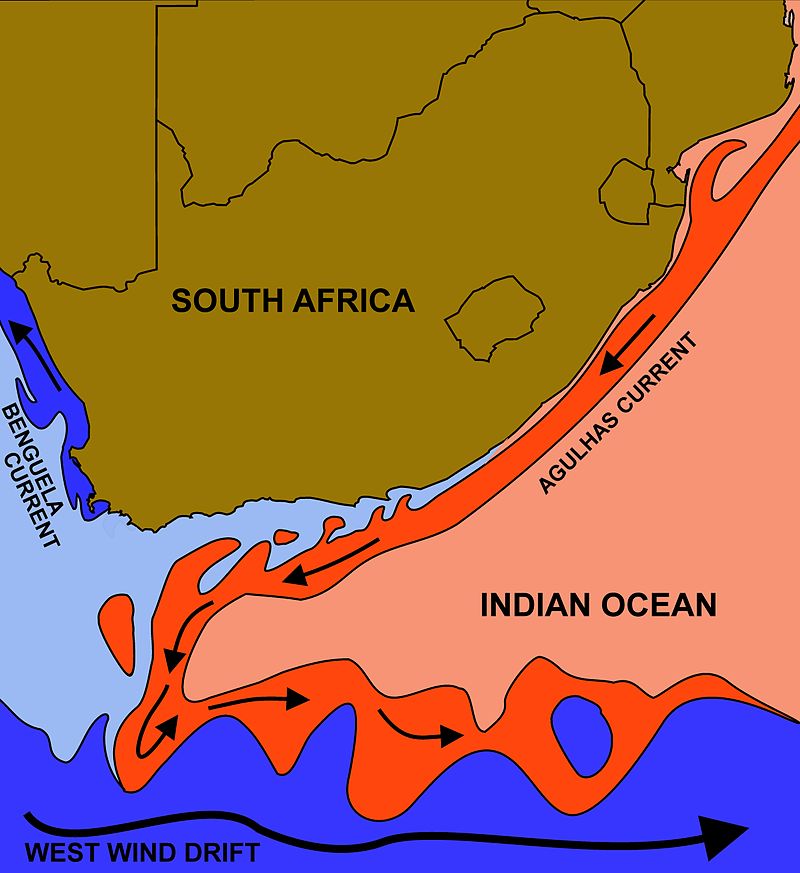
Image from Wikipedia.
To understand the recent results in optics, we need to say a few words about the meaning of the term rogue wave and their possible physical origins. Gigantic waves such as the Draupner wave are not impossible under traditional wave theory, but are so statistically unlikely as to effectively never occur. A rogue wave is therefore usually defined by its likelihood. Ocean researchers use the somewhat arbitrary threshold of “being more than twice the height of the significant wave height,” where the “significant wave height” is four times the standard deviation of the sea elevation. What distinguishes a rogue wave from an ordinary wave, then, is simply whether seeing one is likely.
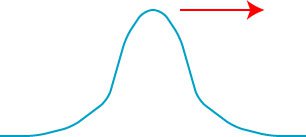
From Greg Gbur's blog.
So what processes can make large waves more likely to occur? The most common explanation relies on so-called nonlinear effects, in which the behavior of a wave is influenced by its size. The simplest possible example of such an effect is a situation where the wave speed depends on its height at a particular point. Suppose, for instance, we start with a simple single-humped wave, as shown above.
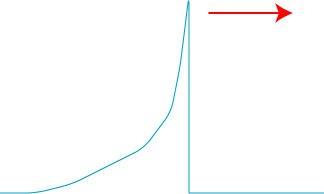
From Greg Gbur's blog.
Let us imagine that the wave moves faster at points where it is taller. The peak of the wave will overtake the tail of the wave to the right and gobble it up, taking it along for the ride. As time goes on, the tall part of the wave will have absorbed everything to its right, and might look as illustrated above.
We end up with what appears to be a “wall of water,” which is extremely telling because mariners have historically described rogue waves this way! This example, however, is oversimplified, and real nonlinear rogue wave effects are expected to be much more complicated.
It is also thought, however, that other, simpler, effects can contribute to and cause rogue waves. For instance, the Southern tip of Africa is known for a high frequency of rogues, and it is suspected that this is due to the collision of two competing currents from either side of the continent, the Benguela Current and the Agulhas Current (see the map, above).
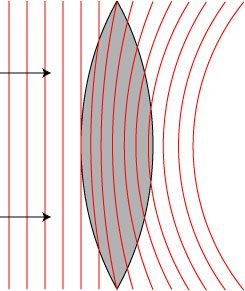
From Greg Gbur's blog.
As waves travel from a region where they are moving along with the current to one where they move against it, they will slow down and their heights can increase dramatically, as illustrated below.
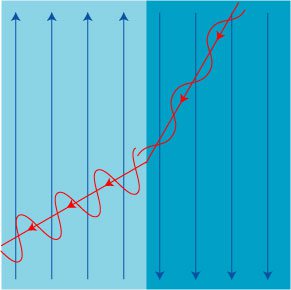
From Greg Gbur's blog.
The shape of the seafloor can also create wave “hot spots.” Just like a lens can focus light waves, seafloor features can alter the direction of water waves, causing them to converge into high-amplitude spots, possibly rogue waves.
It is, in essence, this latter possibility studied in optics in the recent collaboration. The researchers shined a laser beam through a spatial light modulator, a device that essentially “scrambles” the laser beam. (Waves passing over a very rough region of seafloor would be the analogous scrambling for water.) As the beam propagated, it started to interfere with itself, creating regions of constructive and destructive wave interference. With an imaging system, the researchers measured the intensity of the light in a three-dimensional region of space, and they measured the statistical distribution of light intensity in this region.
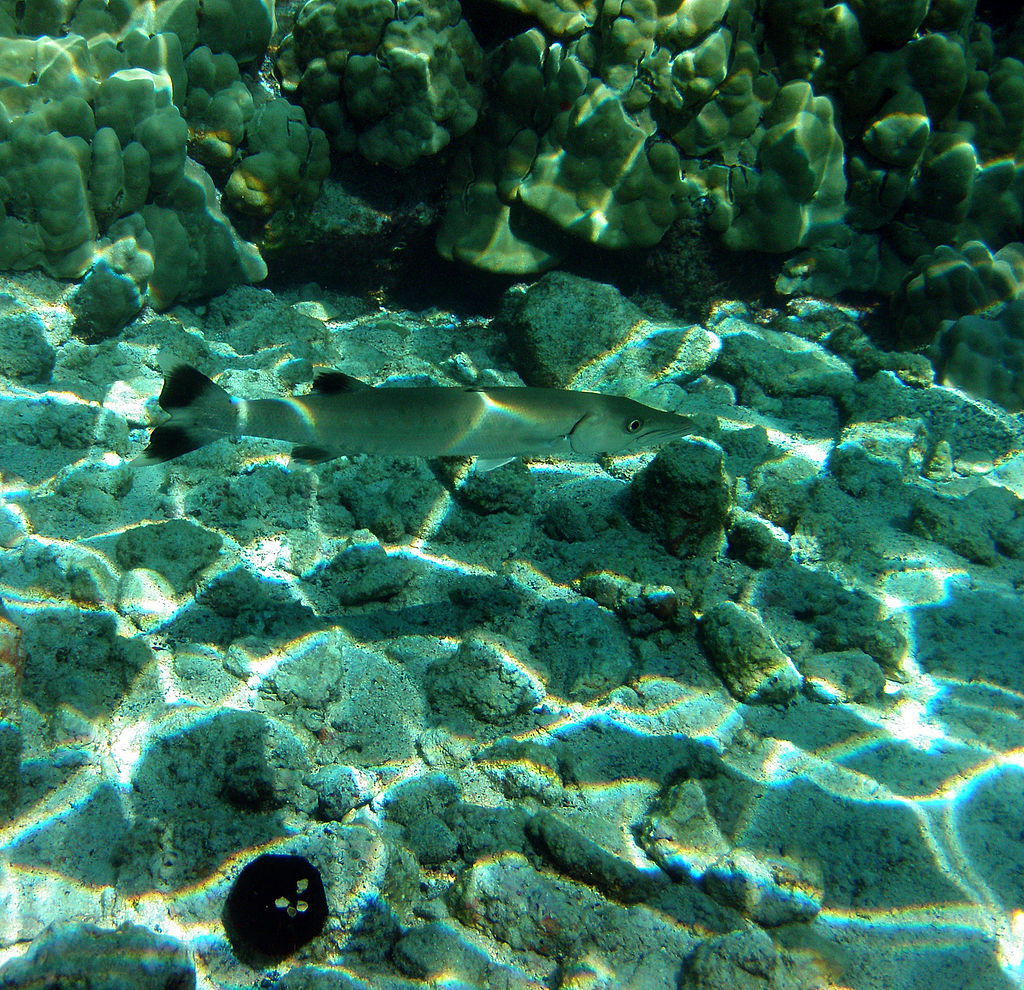
Image from Wikipedia.
When a beam of light gets scrambled, it exhibits two distinct behaviors as it propagates. First, it manifests a network of bright lines, or caustics, which represent the light imperfectly focusing. This is the same sort of effect one sees at the bottom of a swimming pool on a sunny day.
As the light travels further, the wave interference effects become more pronounced and localized, and the light forms a so-called speckle pattern, as seen below.
The researchers studying optical rogue waves investigated the propagation region intermediate between the caustic region and the speckle region, which they dubbed the “optical sea.” An example of their results is shown further below.
They measured the intensity of the propagated light, and from these data reconstructed the actual optical field, the quantity which is most analogous to the wave height of the ocean. In this particular example, they spotted one peak that qualified as a rogue wave, according to the definition given earlier. Overall,they found that the statistical distribution of wave heights suggested that rogue waves occur with a higher probability than previously expected.
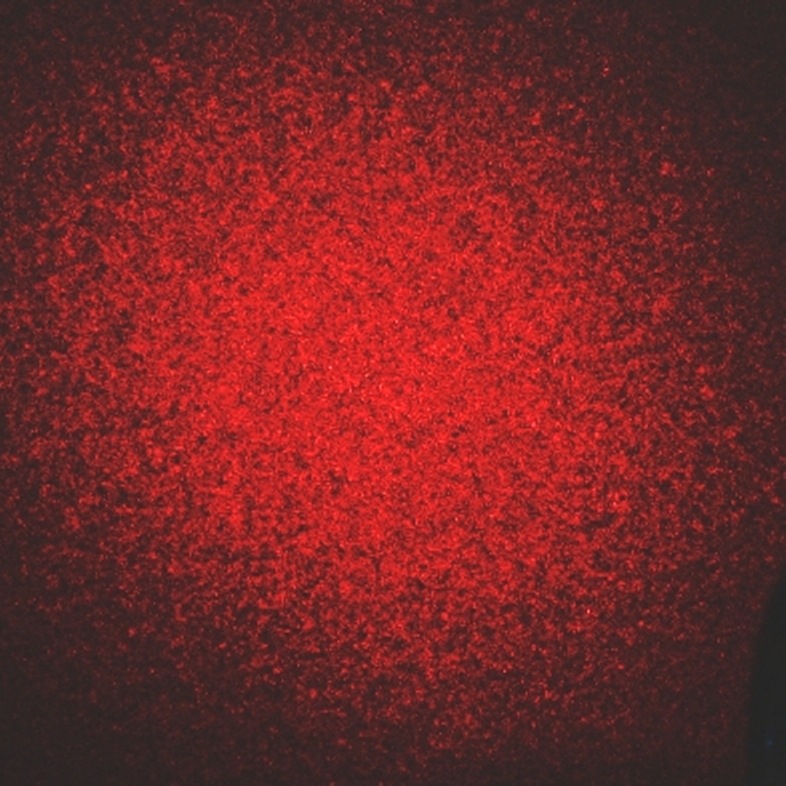
Image from Wikipedia.
The authors justified the use of the term “optical sea” by noting that there is a striking similarity between their optical wave measurements and a typical image of rough ocean wave heights. Their results demonstrate quite clearly that it is possible to see extreme wave events in very simple systems, even in absence of nonlinear effects.
This experiment, although seemingly quite dissimilar to the giant monsters of the ocean, shed light (literally) upon the formation and likelihood of deadly rogues. It indicates that linear processes, like the effects of seafloor features, can play a significant role in rogue formation. In the future, such studies may help determine what regions of the ocean are most likely to produce rogues, and under what conditions.
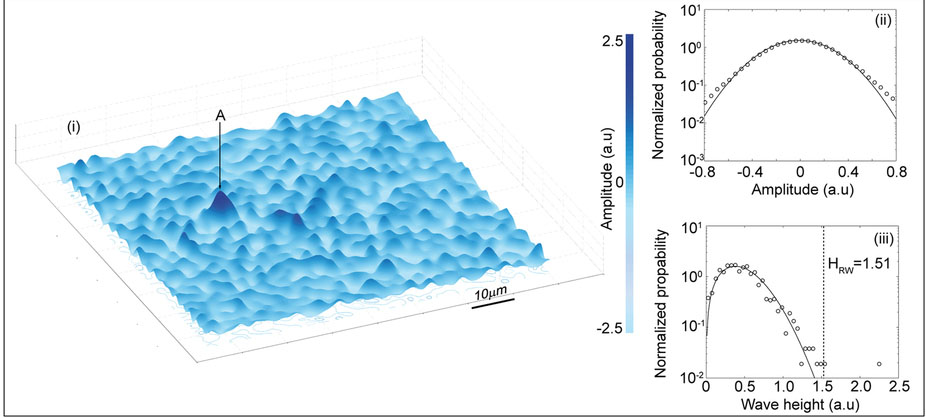
Even if we can’t predict rogue waves, perhaps we can hide from them in some circumstances. My recent feature discusses new ideas about “cloaking” that could potentially reroute waves to protect stationary ocean platforms and buoys.
American Scientist Comments and Discussion
To discuss our articles or comment on them, please share them and tag American Scientist on social media platforms. Here are links to our profiles on Twitter, Facebook, and LinkedIn.
If we re-share your post, we will moderate comments/discussion following our comments policy.