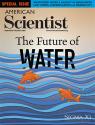
This Article From Issue
September-October 2019
Volume 107, Number 5
Page 276
The 2013 National Medal of Science in Chemistry and 2018 Priestly medalist, Geraldine Richmond is the Presidential Chair in Science and a professor of chemistry at the University of Oregon, and the president of Sigma Xi. Her research focuses on what happens at the surface of water—a crucial location for understanding pollutants and oil spill remediation, as well as a number of biological processes. She spoke to American Scientist’s editor-in-chief, Fenella Saunders, about using high-speed laser pulses to interrogate the surface of water and determine the interactions of chemical bonds there.
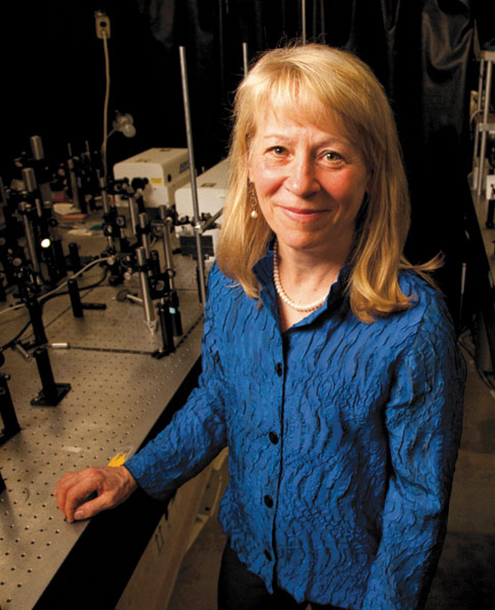
Courtesy of Geraldine L. Richmond
What drew you to surfactants and the water interface as a place to focus in chemistry?
I’ve been fascinated by water for a long time. It has so many special properties. We think of water as an isolated molecule, but it has a gregarious nature and plays with a lot of other water molecules. That’s where a lot of mysteries come in, including what happens at the surface of water. Being able to understand the surface of water and why it has these unique properties of high surface tension, and how that surface tension then affects the bonds—what’s the collective behavior between water molecules at a surface? That question has fascinated me in large part because it has so many environmental implications. Everything that gets into water has to go through the surface. I’m fascinated by trying to understand what’s happening there.
You have designed and conducted experiments with lasers to investigate the water surface. How do those experiments work and what do they telling you about the molecules at the surface of water?
The tricky part about studying any surface, and particularly a water surface, is you really only want those molecules at the very top. You don’t want 100 molecules down. You want the top one, two, three layers.
We use a nonlinear optical method, where we put two laser beams onto the surface to interrogate the molecules that are just on top. Those laser beams are very short pulse, very high intensity, but not so high that they boil the water away—we do try to avoid that—or heat up the water. The point is to induce these molecules on the top layer to polarize, to slosh their electrons around and give us some information about how they’re behaving. In the light that comes off of the surface, we get a little tiny bit of a beam that has all of the information in it.
The information that it carries can only come from the very top layer. It can’t happen in the bulk of water, it can only happen at the surface. That special light gives us information about how water molecules at the surface bond to each other, how a single molecule moves around and vibrates, and when it vibrates and grabs on to somebody else, what that gravity or bonding looks like. If you put something on the surface, then we can measure its properties, too. We call that vibrational spectroscopy, because it’s giving us information about how molecules vibrate and the signature modes of those molecules. It not only detects what’s there, but it tells us how they’re bonded.
But the laser technique doesn’t do everything. That’s why we complement the experiments with theoretical computational methods to get even more information. It’s very powerful to have the two combined.
How can you identify the readout from the lasers as coming from the water surface?
We can distinguish what kind of molecule is there based on what that frequency is. The frequency we get is a spectrum with a series of peaks. The frequency of any of those peaks—the energy of any of those peaks—is characteristic of a kind of bond.
We normally think of water as having OH [oxygen-hydrogen] bonds. OH bonds stretch. It isn’t just one frequency, it can shift a lot. If a single water molecule is isolated at the surface, it’ll have a frequency associated with the OH bonds, but once they start sticking together, that frequency moves further and further into the red, meaning the wavelength is getting longer. If we were going into blue, it would be getting shorter. Going into the red is stronger bonding.
By looking at the kind of signal you get for water, you can tell whether the molecule is isolated at the surface, or if it’s bonded to several other water molecules. Water at the surface has a very interesting behavior that we can detect with this measurement: Some of the molecules have their OH bond sticking out of the water, and others sticking into the water. That gives a particularly interesting kind of a spectrum.
Why do some of those OH bonds stick up or stick down?
It’s not really understood. Even when you do simulations you see it; there’s a desire for some of these water molecules to be bonded like this. When the molecule sticks up, it sets up a very interesting junction. This guy sticking out of the water can then bond to other species. Also, surface tension is high, therefore it’s almost like plastic wrap at the very top layer, but it evaporates. You have to have some freedom there for water molecules to leave, as well as to have stronger bonding.
What we’ve learned from these experiments is you have these guys that straddle the interface. Then you go a bit deeper and they tend to be a bit stronger bonding than this. Then you go into the bulk water, and you have liquid water and a real party going on, where everybody’s grabbing on to different partners and everything else. But it’s very different as they move to the surface and they start to be a little more introverted. I like to say they’re stressed out because they don’t have enough dance partners in the area that want to dance with them. There’s a transition from this bulk party behavior to where you have this more isolated behavior.
Your research into the strength of these bonds at the surface of the water has led to developments in cleaning up oil spills. How does the chemistry work to break up these bonds between the water and the oil?
A lot of what we’ve done in recent years is to put oil on water. That’s where the work was really ground-breaking, identifying these weak bonds between the molecules sticking out of the water and the oil.
The bottom line when you have an oil spill is you want to be able to break it up. You want to break it up into little tiny droplets. But those little tiny droplets of oil, because they’re also in ocean water, need to be stabilized, so they’ll stay as a droplet. The dispersant or the soap that you put on the oil, whether it’s on your laundry or on an oil spill, has to encapsulate that droplet of oil to hold it together. The more effective it is at holding it together, the more likely it will survive long enough to be washed out to the ocean or be biodegraded.
The problem is that the compound that they use now to do that in an oil spill—called Correxit—many of the components in that are safe, but you mix them together and they’re just awful and toxic. Coming up with a better understanding of how to encapsulate oil and what kinds of bonding interactions lead to that stronger encapsulation is really the holy grail of the field, not only for oil spills, but also a lot of other environmental uses.
What we’ve done over the years is to first study very simple soaps and surfactant: how they interface with water and how they are structured. Do they lay down? Do they stand up at the interface? How do they behave, and how is that related to a macroscopic property like measuring the surface tension? Because soaps work effectively to lower the surface tension.
What we’ve been doing most recently is we’re making little droplets and looking at how different soaps stabilize that droplet. What are the properties of a surfactant that contribute to that nanoemulsion being stabilized? It’s a trickier experiment to do, because you still use the two laser beams, but you’re now scattering them off a lot of tiny droplets. Collecting the scattered light just makes it a little bit trickier.
Of course, oil isn’t the only substance coming in contact with water molecules. Can your work also apply to other areas?
There’s a lot out there with regard to what we would call water coming in contact with a hydrophobic surface, hydrophobic meaning water-hating, something like an oil. Even soils have hydrophobicity to them. Anything that we can learn about water next to a hydrophobic medium has implications not only for oil spills or toxic spill cleanup, but also for oil drilling and oil recovery. Having safer surfactants to pull oil out of the earth is really important, too. A lot of the detergents that they pump down into the well to bring up the oil are pretty disgusting. We’re not going to solve all the world’s problems by any means, but we hope to be able to give insights and allow people to predict which methods might be more effective than others, with the tradeoff being what’s safe, and how far do you want to push with regard to effectiveness and safety.
A lot of the work that we do with liquid oil has relevance to anything that’s hydrophobic, which is creating something that’s just easier to work with. But the work also has a lot of implications in biological systems. Your whole body is water, aqueous, next to a cell membrane, and we’ve done some work related to how ions transport across those membranes.
Some of the work that we’re doing with these little droplets relates to drugs, because for some of these nanodroplets, if you put the drug inside of it, it allows delivery to more specific sites. We’re starting some studies where we’re looking at applications related to drug delivery in the pharmaceutical area.
Think about your body. At any moment, you have millions of chemical reactions going on. They’re giving off heat and taking in heat, but your temperature doesn’t change. That’s because it stays constant, because there’s so much water in your body that absorbs a tremendous amount of heat, but doesn’t change its temperature very much. To me that’s as mysterious as anything else.
Was there a moment in your career that was really challenging that you were able to overcome and move beyond?
When I was at Bryn Mawr College, first starting in the early stage of assistant professorship, my students and I built my first laser. It was so exciting. We came up with the first set of experiments to do in collaboration with another faculty member. I had a couch in my office, and I was taking data around the clock, 24 hours, and I’d take a nap in between. I was just so excited about everything, about getting this great data and showing that the experiments worked, and that the laser worked.
There were samples being prepared for me, and I was getting ready to write up a paper, and then I discovered that the samples had an artifact. I was studying an artifact, not what I thought I was studying. That was just devastating, because I’d worked so hard to get the laser up and going. All these students and I just—it’s just really hard. As I tell people, normally I have a lot of energy. I’m up and going all the time. That was the one morning I didn’t want to get out of bed, once I discovered that the day before. I just didn’t know how to go on.
But I got up and did my usual routine of going for my long run in the morning. I came back and decided it was time to shift fields and use the lasers in another kind of experiment. And that’s when I came up with this idea to use the lasers to study surfaces. That’s where I am today.
That one thing that I thought was just devastating was what I needed to then become the one driving the experiments, designing them in those initial years, and figuring out what to do rather than relying on someone else. It was a good lesson for starting out, because you need to know everything about your experiments.
The wonderful thing about this particular area of science is that students gain a lot of training in optics, chemistry, surfactants, computer programming, and machining, and they see how the different fields relate. That’s why I like it. You’re not just stuck in one little silo.
An audio interview is available below:
American Scientist Comments and Discussion
To discuss our articles or comment on them, please share them and tag American Scientist on social media platforms. Here are links to our profiles on Twitter, Facebook, and LinkedIn.
If we re-share your post, we will moderate comments/discussion following our comments policy.