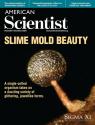
This Article From Issue
November-December 2024
Volume 112, Number 6
Page 329
Xin Zhang received her doctorate in mechanical engineering, working on tiny devices that convert back and forth between electrical inputs and physical work, called microelectromechanical systems (MEMS). But she started out in materials science, with an interest in solid and fluid mechanics. Zhang, now a distinguished professor of engineering at Boston University, credits collaboration across engineering and other disciplines with finding the connections that have helped her make functional and innovative devices. Zhang now focuses on metamaterials, which are engineered to have mathematically optimized structures, to create devices that can, for instance, reduce noise without blocking airflow or heat, or that can improve the signals in medical imaging. Metamaterials, she says, can be made to any size or shape, because their function depends only on their structure, not their chemical constituents. Zhang was the recipient of the Walston Chubb Award for Innovation at the 2023 International Forum on Research Excellence (IFoRE), and spoke with editor-in-chief Fenella Saunders after the conference about her work. (This interview has been edited for length and clarity.)
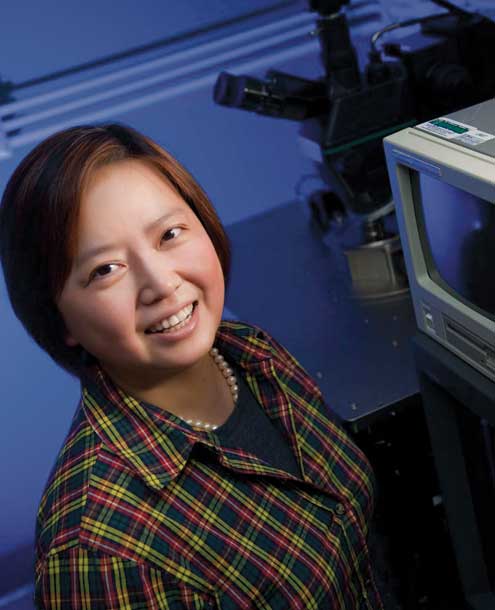
Courtesy of Xin Zhang/Boston University.
What are microelectromechanical systems (MEMS)?
MEMS are a set of sensors, actuators, and detectors. Cars and phones have tons of MEMS that are part of the structure of those devices, such as pressure sensors, gyroscopes, infrared detectors, and many others. When you rotate your phone and it immediately adjusts, that’s a sensor at work inside. When you drive a car in the dark, you might have infrared sensors that allow you to see a deer by the road. The size of MEMS is also just about right for use at the micro-level in the body, for biomedical microdevices, biosensors, and laboratory-on-chip applications, among others. You can also have actuators, such as motors, generators, or rockets. You can have a rotor just a few millimeters in diameter, capable of spinning up to millions of revolutions per minute, suitable for micro- or nano-robots and other dynamic systems.
How do you create metamaterials?
Metamaterials are mathematically designed, artificially engineered materials. We can customize the electromagnetic, mechanical, and acoustic properties by designing and manufacturing things through technologies such as 3D printing. You can think of the units that are put together to make metamaterials as something similar to meta-atoms, in that their size is smaller than the wavelength of the application. You can think of sub-wavelengths of small units.
How can metamaterials be used to help with noise control?
Noise is everywhere. I’m from a big city and I like it, but no matter how fancy your building, you can hear noise. Usually, noise is controlled by covering it, like the thick barriers you see when you’re driving on a highway. But what if you want to allow heat and light to pass through? Now, we can reduce noise, and we also can have transparency and openness. For example, if you think about buildings and HVAC systems, or data centers, we can allow airflow with reasonable noise reduction.
I worked with a talented student on acoustic metamaterials. In the beginning, we failed many times. We were thinking too aggressively about ultrasound, about clinical medical ideas, and it didn’t work. But there were one or two successful ideas that we could potentially publish. I was chatting with him and other students and saying that I know a lot of friends who have problems sleeping. They have a stressful day, and then they’re very sensitive to noise, so they’ve bought all sorts of materials to attach to their windows and walls. That kind of inspired us to think about, what can we design that will stop noise but allow people to see through? We started thinking about something such as a “smart” window, and we were able to come up with a rough idea of a kind of dome or a ring with a very open central area. We mathematically designed these ring structures with six embedded channels that manipulate acoustic waves. Simply speaking, when you hear a sound outside of the ring, the sound has two paths. One is to go through this central open area and you hear it directly. The other is to go through these six channels. If you design it correctly, these two paths will meet on the other side. What goes through the ring will have a phase change of 90 degrees, and it will cancel out on the other side. It’s not being absorbed or covered, it’s being cancelled. If you really have something annoying, like an air conditioner that’s driving you crazy, and you measure its frequency at say 1,000 hertz, this structure works very well for something primarily at a single frequency.
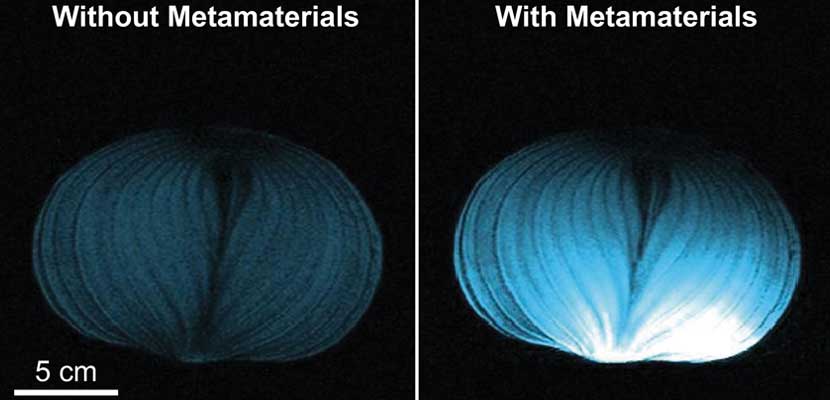
Courtesy of Xin Zhang/Boston University.
What is the time frame for using metamaterials to cancel noise?
In the near term, I’d say it’s going to be something direct such as HVAC systems or other fans, simple cases with one frequency. But fans have applications from hundreds of meters wide to very small, such as dedicated fans used in brain surgery. Even dentists have contacted me about this metamaterial. They’re all worried about noise. A lot of people also are interested in uses for cars. Ten different car companies have contacted me, and I thought they would all be asking for the same thing, but they have 10 different cases. But tunable devices that can deal with multiple signals or frequencies are challenging. The long-term case, which people care about deeply, is to insert this material into building architecture. But that’s going to be a long road.
But if you want me to make a prediction, you’re going to see a lot of applications for acoustic metamaterials. Not just for sound silencing but also sound manipulation. You and I might be in a physical conference, in an open area, and you want to make an announcement, but you only want to reach the target audience. Or if you’re driving a car with your sister and she’s listening to annoying music, you can deal with that. Sound manipulation and wave propagation are going to be very popular.
I’ve learned from the general scientific community about applications. We’ve been contacted by hundreds of companies, and very directly, people say: “I’m bad at math, can you build a library? With the popularity of 3D printers, if you give me a library, I can print something for myself.” That kind of comprehensive documentation is what we get asked about the most.
Do metamaterials have any limits on their sizes or shapes?
The metamaterial is not shape-limited and is not material-specific; they can be free-form and flexible. If you deal with harsh or special environments, you can use any special materials through 3D printing or other manufacturing techniques. Metamaterials are made of unit cells that can be any shape. Depending on the application, you can use an array of them to make large structures.
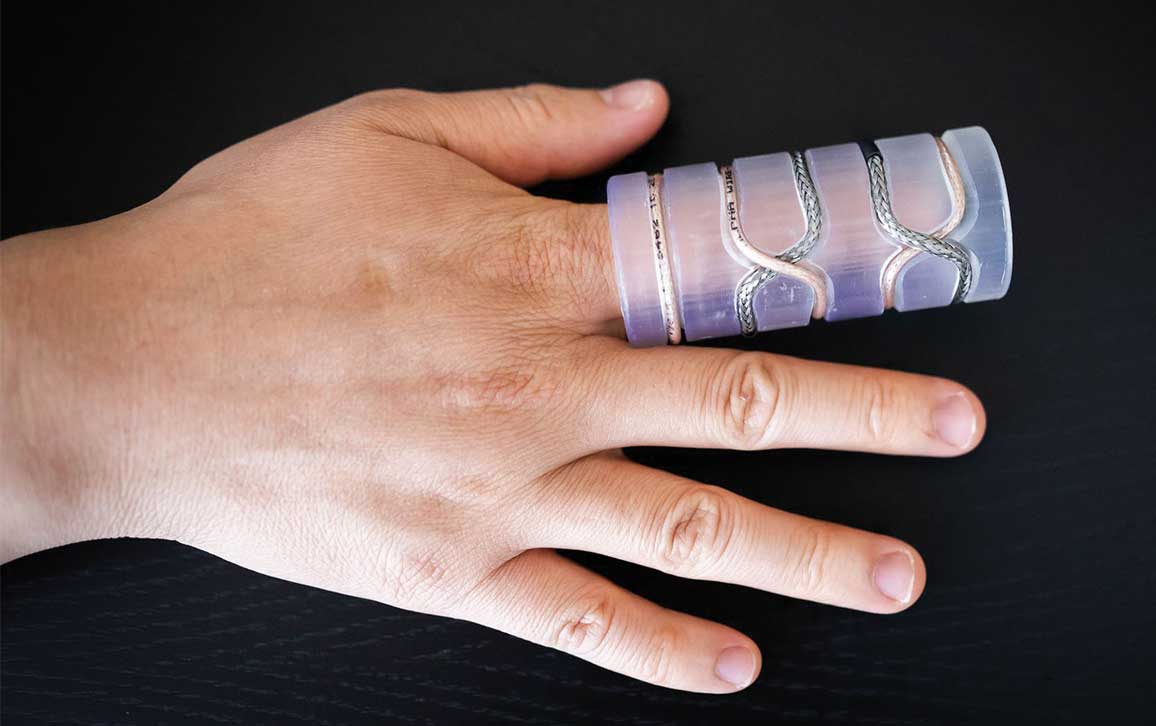
Courtesy of Xin Zhang/Boston University.
What other applications are you exploring for metamaterials?
I am collaborating with a radiologist on magnetic metamaterials for magnetic resonance imaging (MRI). Radiologists tell me the most important thing to them is a good signal-to-noise ratio, which allows them to decrease the time it takes to scan each patient. The way MRI works, the radio frequency waves excite the patient, then the patient releases the absorbed energy, and at that point, the metamaterials near the patient interact with those waves and enhance the signal going back. The structure of the material is mathematically designed to optimize wave manipulation. That enhancement will give you a better signal-to-noise ratio. People aren’t comfortable during long MRI scanning times, in the cramped and noisy environment when they can’t move anything, so time is important. It could also let you use ultra-low-field MRI, which is portable in the field and less expensive for developing countries.
How can metamaterials improve other types of imaging?
MEMS-based detectors can sense infrared for seeing in the dark or in low visibility conditions, like for firefighters, but they need to be more accurate. That’s where electromagnetic metamaterials were able to play a role, from a design viewpoint. This kind of thermal imaging allows you to see anything you would want to see at a certain frequency, such as for safety scans. You could also go the opposite way; mathematically, you can do more optimization and have the best absorbers. Then you can demonstrate a unit cell with manipulation and detection at certain bands of the electromagnetic spectrum for even more optic and photonic applications.
What are your next applications?
I’m a big fan of meaningful, practical applications, that improve quality of life and have societal benefit. Instead of creating something new I’d like to focus and push as far as possible so that when I retire, I can see a result that is something truly beneficial. I can point to this and say, I made it and it helps people. As I get older, I care more and more about more deeply meaningful applications. AI technology is my current example. I never thought I would get into machine learning or deep learning. But in recent years, because of my collaboration on MRI and metamaterials, that gave me an opportunity to do some blue-sky research. Can we not only improve the performance of a clinical MRI, but in the next 20 years, can we also develop a portable, low-cost, low-field MRI? Can we push every limit of the hardware and metamaterials to try to answer that question? That’s where I picked up the AI part. There was no way, if I didn’t have AI technology to help with this complex system, that we could push toward low-field or even ultra-low-field MRI. Maybe in five or 10 years, half my research will be AI. Who knows? I like pursuing something that isn’t yet too well-defined into the future.
American Scientist Comments and Discussion
To discuss our articles or comment on them, please share them and tag American Scientist on social media platforms. Here are links to our profiles on Twitter, Facebook, and LinkedIn.
If we re-share your post, we will moderate comments/discussion following our comments policy.